The Particle World
What are neutrinos
telling us?
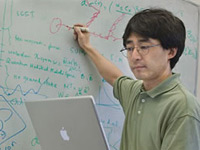
View the Video
Ubiquitous, elusive and full of surprises, neutrinos are the most mysterious of the known particles in the universe. They interact so weakly with other particles that trillions of them pass through our bodies each second without leaving a trace. The sun shines brightly in neutrinos, produced in the internal fusion reactions that power the sun. These reactions produce neutrinos of only one kind, but they mysteriously morph into two other kinds on their way to earth. Neutrinos have mass, but the heaviest neutrino is at least a million times lighter than the lightest charged particle.
The existences of the neutrion’s tiny nonzero mass raises the possibility that neutrinos get their masses from unknown physics, perhaps related to unification. Detailed studies of the properties of neutrinos—their masses, how they change from one kind to another, tell us whether neutrinos conform to the patterns of ordinary matter or whether they are leading us toward the discovery of new phenomena.
Tools for a scientific revolution
The discovery that neutrinos have mass opens a window on physics beyond the Standard Model. The Standard Model cannot accommodate neutrino masses without the introduction of new particles, which themselves raise new questions. In fact, the size of the neutrino masses is consistent with expectations from unified theories that require the new particles for the unification itself.
The most pressing question about neutrinos involves how many different kinds there are. Results from the Liquid Scintillator Neutrino Detector experiment suggest that there may be more than the canonical three families. If so, that would require a major revision of current understanding. The Mini-BooNE experiment, now running at Fermilab, already hints that multiple kinds of neutrinos are likely to exist. The first results from Mini-Boone conclusively show that the LSND results could not be due to simple neutrino oscillation, a phenomenon in which one type of neutrino transforms into another type and back again.
Even if there are only three kinds of neutrinos, questions remain. What generates the neutrino masses and what are their values? Are neutrinos their own antiparticles? How do different kinds of neutrinos mix? Answering these questions requires precision measurements of the neutrino masses and mixings. Observations of neutrinos by the Super-Kamiokande experiment in Japan and the SNO experiment in Canada provided the first conclusive evidence for neutrino masses and mixing. Physicists are now studying neutrino mixing at the NuMI/MINOS program and the CERN-to-Gran Sasso long-baseline neutrino program. The neutrino beam at JPARC is being developed in Japan. In the longer-term future, experiments such as Daya Bay in China, Double CHOOZ in France, T2K in Japan and the NuMI Off-Axis Electron Neutrino Appearance Experiment ( NOvA ) at Fermilab, may tell us if a measurement of CP violation in the neutrino sector is feasible. Then researchers might use a neutrino superbeam or neutrino factory to search for it. The detector in such an experiment could also search for proton decay, if located deep underground in a facility such as the proposed Deep Underground Science and Engineering Laboratory.
Accelerator and reactor oscillation experiments measure mass differences. The masses themselves must be determined by different methods. Neutrinoless double beta decay experiments such as EXO and the planned Majorana experiment can be used to measure the electron neutrino mass to ~0.01eV, if neutrinos are their own antiparticles. The observation of neutrinoless double beta decay would have far-reaching consequences, raising the possibility that the matter and antimatter could have transformed into each other in the early universe.
- Last modified
- 04/28/2014
- email Fermilab