The Particle World
Why are there so many kinds of particles?
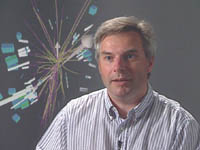
View the Video
We have discovered three families of quarks and leptons, families of fundamental particles that differ only in their masses, which range from less than a millionth of the mass of an electron to the mass of an atom of gold. Just as quantum mechanics led to an understanding of the organization of the periodic table, we look to new theories to explain the patterns of elementary particles. Why do three families of particles exist, and why do their masses differ so dramatically?
Current investigations focus on developing a detailed picture of the existing patterns in the particle world. Remarkable progress has been made, especially in characterizing the quarks. But why are the patterns for leptons and quarks completely different? Detailed studies of quarks and leptons at accelerator experiments will provide the clearest insight into these issues.
Tools for a Scientific Revolution
Physicists have so far identified 57 species of elementary particles. In particular, the Standard Model contains quarks and leptons, grouped into three families that differ only in their masses. Why the pattern of particles is repeated three times with enormous variations in mass but with other properties seemingly identical is an open question. Quantum physics has shown that three families are the minimum necessary to accommodate CP violation in the Standard Model. Such CP violation is necessary for matter to predominate over antimatter in the universe, but its effects observed so far are insufficient to explain this predominance. The current program of experiments focuses on developing a detailed understanding of the existing patterns and searching for signs that the patterns of the three families are not identical.
Even through the Tevatron was shut down, the CDF and DZero experiments at the Tevatron, which recorded collisions until September 2011, continue to provide information on the top quark and check if its enormous mass gives it a special role in the particle world. The BaBar and Belle experiments at SLAC and KEK used their data samples, containing millions of b and c quarks, as well as τ leptons, to make precision measurements of the masses and decay modes of all of these objects, in order to look for subtle deviations from the predicted patterns of their decays. The third- generation particles—top, bottom and tau—offer the best hope for discovery, because their large masses allow them to couple most effectively to undiscovered physics.
BaBar and Belle could study only two types of B mesons, bound states of the bottom quark with up or down quarks. However, many theories suggest significant effects in the bound state with the strange quark, Bs. Physicists at the Tevatron made important measurements on the properties of the Bs meson and discovered the fastest matter-antimatter oscillations ever observed. The LHCb experiment explores the Bs meson with far greater precision.
Properties of individual quarks are experimentally difficult to study, because they are always bound to other quarks. Lattice Computational Facilities offer great promise for the calculation of the effects of the strong interactions. As an example, lattice calculations will provide sufficient precision to extract quark parameters, such as those that describe flavor mixing, from the experimental data.
Neutrinos have opened a surprising new window on the physics of lepton generations, since neutrino masses are not necessary in the Standard Model. The presence of neutrino masses may be telling us something about the physics beyond the Standard Model. The decay properties of the light leptons, the electron and the muon, may also hold surprises. Fermilab pursues an ambitious research program to explore the secrets of these particles in more detail.
- Last modified
- 04/28/2014
- email Fermilab