The Birth of the Universe
How did the universe come to be?
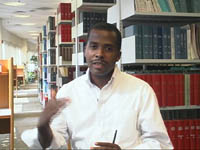
View the Video
According to modern theories of cosmic evolution, the universe began with a singular explosion, followed by a burst of inflationary expansion.
To understand inflation requires breakthroughs in our understanding of fundamental physics, quantum gravity and the ultimate unified theory.
Although inflationary conditions are too high in energy to reproduce on Earth, we can observe their signatures, transmitted over the eons by their imprint on the relic matter we can still detect from that era.
Following inflation, the conditions of the early universe were still so extreme they could combine elementary particles into new phases of matter. As the universe expanded and cooled, transitions took place as matter changed from one phase to another, like steam condensing into water. Some of these phase transitions may have been the most dramatic events in cosmic history, shaping the evolution of the universe and leaving relics observable today. Cosmic phase transitions could be recreated in high-energy accelerator experiments.
Tools for a scientific revolution
According to current theories of cosmic evolution, the universe begins with an “initial singularity,” a point where all known laws of physics break down. This singularity produced a delicately balanced universe, like a pencil so precisely balanced on its point that it stays upright for 14 billion years. How did the universe reach such a state? How did it get to be so old? Why has it not blasted even further apart or collapsed back on itself?
For the last two decades, the theory of cosmic inflation has offered a compelling explanation of the start of the big bang. According to this theory, an early phase of accelerated expansion gave rise to the balanced universe we see today. Cosmic inflation is the hand that balanced the pencil on its point. As a by-product, it also produced the seeds that evolved into stars, galaxies, clusters of galaxies and other structures in the universe.
Cosmic inflation presents challenges related to the fundamental questions in this report. One possibility is that cosmic inflation originated with a form of dark energy, akin to the dark energy observed today. If so, what kind of matter produced it? Does this form of matter play a role in unification? How does it relate to extra dimensions? Even more radical is the possibility that space and time changed their nature at the start of the big bang. Does string theory smooth the initial singularity? Which model did nature really choose?
At present, measurements of fluctuations in the cosmic microwave background (CMB), especially from WMAP, provide the best evidence in favor of inflation. Constraints on cosmic parameters, such as the curvature of the universe, and the nature of the cosmic structure, are in broad agreement with the predictions of inflationary theory. Eventually, measurements of the polarization of the CMB may permit the detection of the signatures of gravitational waves produced during the epoch of inflation, which could provide information about the nature of the scalar field that produced inflation.
After the big bang, the universe expanded and cooled to reach its present state. Along the way, the universe passed through a series of phase transitions in which various particles froze out, as water turns to ice as it cools. These phase transitions drove some of the most important epochs of cosmic history. For example, a phase transition may be what drove cosmic inflation. Phase transitions might produce “cosmic defects,” such as strings and texture and other exotic forms of matter, that could explain ultra-high-energy cosmic rays, dark matter and perhaps even dark energy.
Experiments at the LHC will continue to illuminate the electroweak phase transition, where most of the known particles acquired their masses. Better understanding of this phase transition will allow scientists to push closer to the big bang itself. Indeed, it is likely that the electroweak phase transition is the ultimate source of the matter-antimatter asymmetry we see in the universe today. Discoveries of new particles and new interactions will illuminate this story and determine if it is correct. Moreover, the account of cosmic evolution must incorporate any discoveries of new symmetries or new dimensions.
Currently, the most intensely studied cosmic phase transition is connected with quantum chromodynamics (QCD), the theory of the nuclear force. During the QCD phase transition, the baryonic matter in the present universe condensed from a plasma-like state of quarks and gluons. The Relativistic Heavy Ion Collider (RHIC) facility at BNL is currently creating collisions of heavy ions to study quark-gluon plasma; the ALICE experiment at the LHC investigates the quark-gluon plasma at higher energies and temperatures. The Lattice Computational Facilities will enable calculations furthering the understanding of the RHIC data and the conditions during this epoch in the evolution of the early universe.
- Last modified
- 04/28/2014
- email Fermilab