Quantum science and technology
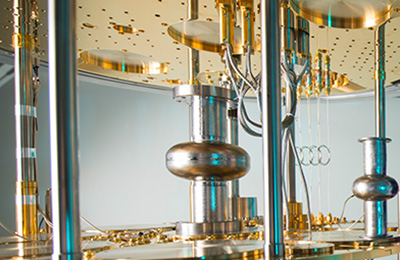
Quantum computing has the potential to take on the most formidable calculations in particle physics, calculations that are, it is not an exaggeration to say, otherwise impossible. Fermilab is pursuing a program to leverage this powerful technology to solve problems in data analysis and theoretical calculations. High-energy physicists are not new to devices that operate using quantum hardware systems, and they are now extending their expertise in sensor and accelerator technology for quantum software and computing. The Fermilab Quantum Institute enables the laboratory to bring these and other quantum projects together and to attract new people and new ideas.
Fermilab leads the Superconducting Quantum Materials and Systems Center (SQMS), one of five national centers to bring about transformational advances in quantum information science as a part of the U.S. National Quantum Initiative. The SQMS Center has the ambitious goal of building and deploying a beyond-state-of-the-art quantum computer as part of an international collaborative effort involving 20 partner institutions. Learn more about the partnerships and goals of the SQMS Center.
- Last modified
- 04/21/25
- email Fermilab