Explain it in 60 seconds: quantum entanglement
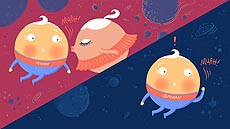 |
Through "spooky action at a distance," the properties of two systems remain correlated even after they are separated. Image: Sandbox Studio |
Quantum entanglement happens when two systems — such as two particles — interact. They develop correlations between their properties that are maintained even after they are separated by large distances in space. An observer measuring one system could perfectly predict the corresponding measurements of a second observer looking at the other system far, far away.
An example of an interaction that generates entanglement is the decay of a particle into two other particles: Due to conservation of momentum, the decay products must be entangled in a state where their momenta are correlated. If we measure the momentum of one particle, we know the momentum of the other.
Albert Einstein disparaged the possibility of this prediction, calling it "spooky action at a distance," but Erwin Schrödinger, and later John Bell, recognized it as an essential feature of quantum mechanics.
Although entanglement doesn't allow communication faster than the speed of light, it can be used to "teleport" a perfect quantum copy of an object (although one must destroy the original). Recent experiments have teleported quantum states well over 100 kilometers.
Entanglement isn't just a feature of esoteric experiments. Typical states of matter we encounter all the time are characterized by a large degree of entanglement. In fact, entanglement between objects and their environment is responsible for the emergence of the familiar classical world from counterintuitive quantum laws. The organization of entanglement in matter can also contain a great deal of interesting physical information.
More speculative recent work has suggested that entanglement might be the thread that stitches together different regions of spacetime in quantum gravity. Entanglement between different objects might even generate a wormhole in spacetime that connects them.
—James Sully, SLAC National Accelerator Laboratory
Read similar articles in the symmetry archive.
|